Nuclear Fission vs. Nuclear Fusion: Unlocking the Power of the Atom
When it comes to unlocking the extraordinary power of the atom, two processes take center stage: nuclear fission and nuclear fusion. While both involve the manipulation of atomic nuclei, they differ significantly in terms of energy release, applications, and their potential to shape the future of energy generation.
Nuclear fission, the process that powers current nuclear reactors, involves the splitting of large atomic nuclei into smaller ones. This releases a tremendous amount of energy in the form of heat and radiation. The controlled chain reactions of fission reactions not only generate electricity but also play a vital role in nuclear weapons.
On the other hand, nuclear fusion, the process that powers the sun and stars, fuses light atomic nuclei together to form a heavier nucleus, releasing even more energy in the process. Scientists and researchers worldwide are working tirelessly to replicate this process on Earth, aiming to harness the immense power of fusion as a clean and abundant source of energy.
In this article, we will delve into the fascinating world of nuclear fission and fusion, exploring their differences, potential benefits, and the challenges they pose. Join us as we unravel the mysteries of the atomic realm and uncover the possibilities that lie within.
Understanding Nuclear Fission
Nuclear fission is a process that involves the splitting of atomic nuclei, specifically those of heavy elements such as uranium or plutonium. When a nucleus absorbs a neutron, it becomes unstable and splits into two smaller nuclei, along with a few neutrons and a large amount of energy. This energy is primarily released in the form of heat, which can be harnessed for various applications, most notably in electricity generation. The fission process is the cornerstone of current nuclear power technology, and understanding its mechanics is crucial for comprehending its implications on energy production and safety.
The fission reaction can be initiated in a controlled manner within a nuclear reactor. When a heavy nucleus fissions, it releases several neutrons that can go on to induce fission in nearby nuclei, creating a chain reaction. This chain reaction is carefully managed through the use of control rods, which absorb excess neutrons and regulate the rate of fission. By maintaining a stable reaction, nuclear reactors can produce a consistent output of energy, which is then converted into electricity through steam turbines. The ability to control this process is what makes nuclear fission a viable source of power in modern energy systems.
However, while fission is a powerful method for generating energy, it also comes with significant challenges and concerns. The byproducts of fission include radioactive isotopes, which pose long-term storage and environmental risks. Additionally, the potential for catastrophic accidents, such as those seen at Chernobyl and Fukushima, raises serious questions about the safety of nuclear power. Understanding both the capabilities and the hazards of nuclear fission is essential for evaluating its role in the future of energy production.
The Process of Nuclear Fission
The process of nuclear fission begins with the absorption of a neutron by a heavy nucleus, such as uranium-235 or plutonium-239. When the neutron is absorbed, the nucleus becomes highly unstable and undergoes deformation. This deformation causes the nucleus to split into two smaller nuclei, often referred to as fission fragments, and releases additional neutrons in the process. The energy released during this reaction is a result of the conversion of mass into energy, as described by Einstein’s famous equation, E=mc². The fission process is characterized by a significant release of energy, typically in the range of 200 MeV (million electron volts) per fission event.
Once the fission fragments are produced, they are typically isotopes of lighter elements, along with the released neutrons. The neutrons can then initiate further fission reactions if they collide with other fissile nuclei, leading to a self-sustaining chain reaction. To maintain this chain reaction in a controlled manner, nuclear reactors are equipped with various components designed to regulate the number of free neutrons available in the system. Control rods, made of materials like boron or cadmium, can be inserted or withdrawn from the reactor core to absorb neutrons and slow down the reaction when necessary.
The overall efficiency of a nuclear fission reaction is influenced by several factors, including the type of fuel used, the configuration of the reactor, and the moderation used to slow down the released neutrons. Commonly, water is used as a moderator to reduce the speed of neutrons, increasing the likelihood of further fission events. The heat generated from these reactions is then used to produce steam, which drives turbines to generate electricity. Understanding the intricate details of this process is essential for ensuring the safe and efficient operation of nuclear power plants.
Applications of Nuclear Fission
Nuclear fission has several significant applications, with electricity generation being the most prominent. Nuclear power plants utilize the heat produced from fission reactions to convert water into steam, which drives turbines that generate electricity. This method of energy production is highly efficient and has the potential to produce large amounts of electricity with lower greenhouse gas emissions compared to fossil fuels. As countries strive to transition to cleaner energy sources, nuclear fission remains a critical component of many national energy strategies.
Beyond electricity generation, nuclear fission is also integral to military applications, notably in the development of nuclear weapons. The energy released from fission reactions is harnessed to create explosive devices, with the most notable examples being atomic bombs. The destructive power of these weapons has led to significant political and ethical discussions surrounding the proliferation of nuclear technology and the need for international treaties to prevent nuclear warfare. The dual-use nature of nuclear fission—beneficial for power generation yet destructive for warfare—poses complex challenges for global security.
Additionally, nuclear fission has applications in medical fields, particularly in cancer treatment. Radioisotopes produced through fission reactions are used in various diagnostic and therapeutic procedures. For instance, certain isotopes can target cancerous cells, delivering localized radiation therapy while minimizing damage to surrounding healthy tissues. The versatility of nuclear fission in both civilian and military domains illustrates its far-reaching impact on society, highlighting the need for responsible management and regulation of nuclear technologies.
Advantages and Disadvantages of Nuclear Fission
Nuclear fission presents several advantages that make it an attractive option for energy production. One of the primary benefits is its high energy density; a small amount of nuclear fuel can generate a significant amount of energy compared to fossil fuels. This means that nuclear power plants require less fuel and produce less waste on a per-unit-of-energy basis. Additionally, nuclear fission generates electricity with minimal greenhouse gas emissions, making it a cleaner alternative to coal or natural gas plants. This characteristic positions nuclear fission as a key player in efforts to combat climate change and transition to sustainable energy sources.
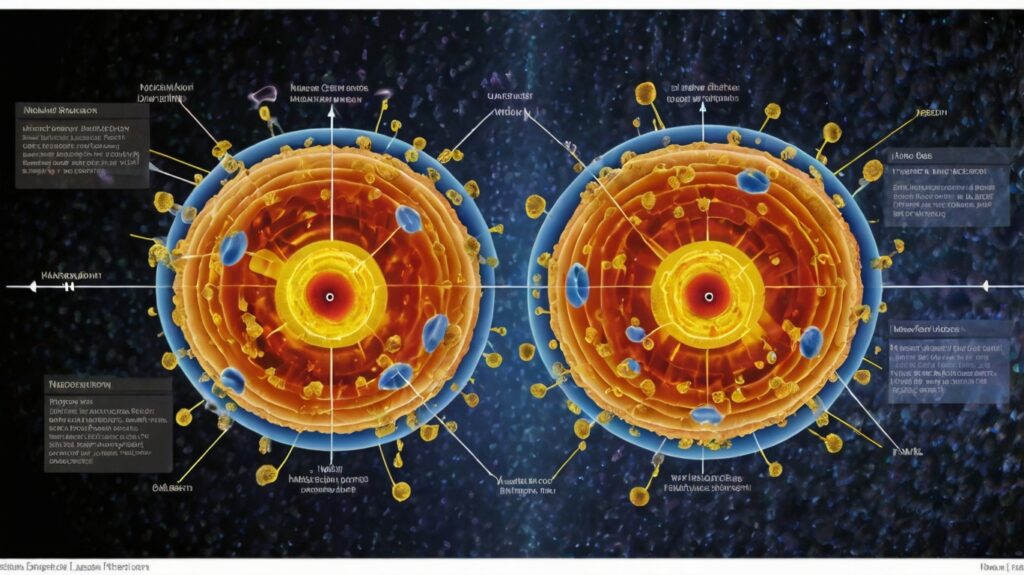
However, the disadvantages of nuclear fission cannot be overlooked. The radioactive waste produced from fission reactions poses serious environmental and health risks. Disposing of this waste safely is a critical challenge, as it remains hazardous for thousands of years. Current storage solutions, such as geological repositories, are still under development and face significant public opposition. Moreover, the potential for catastrophic accidents, as seen in historical events like Chernobyl and Fukushima, raises questions about the safety of nuclear power. The consequences of such disasters can have long-lasting effects on human health and the environment.
Additionally, the proliferation of nuclear technology for military purposes presents ethical dilemmas and geopolitical challenges. The dual-use nature of nuclear fission means that advancements in power generation technology can also lead to advancements in weapons development. This has prompted international efforts to regulate nuclear materials and prevent the spread of nuclear weapons. Balancing the benefits of nuclear fission for energy generation with the risks associated with its military applications is a complex issue that requires careful consideration and robust policy frameworks.
Understanding Nuclear Fusion
Nuclear fusion is the process in which two light atomic nuclei combine to form a heavier nucleus, releasing a tremendous amount of energy in the process. This is the same reaction that powers the sun and other stars, where hydrogen nuclei fuse to create helium. The energy produced through fusion is significantly greater than that from fission, making it a highly sought-after source of power for future energy generation. One of the most promising aspects of fusion is that it relies on isotopes of hydrogen, such as deuterium and tritium, which are abundant and can be extracted from seawater and lithium.
The conditions necessary for fusion to occur are extreme, requiring both high temperatures and pressures to overcome the electrostatic repulsion between positively charged nuclei. In stars, this is achieved due to the immense gravitational forces present, while on Earth, scientists attempt to replicate these conditions using advanced technologies. Various approaches are being researched, including magnetic confinement, as seen in tokamaks, and inertial confinement, which uses lasers to compress fuel pellets. Understanding these processes is crucial for developing viable fusion energy systems.
The potential benefits of nuclear fusion are vast. Unlike fission, the primary byproducts of fusion are neutrons and helium, which means that the environmental impact is significantly lower, as there are no long-lived radioactive waste products. Furthermore, fusion has an almost limitless fuel supply, making it a sustainable option for meeting the world’s growing energy needs. Despite these advantages, achieving a controlled fusion reaction on Earth remains one of the greatest scientific and engineering challenges of our time.
The Process of Nuclear Fusion
The process of nuclear fusion begins when two light nuclei, typically isotopes of hydrogen, approach each other closely enough for the strong nuclear force to overcome their electrostatic repulsion. As the nuclei collide, they must be heated to extremely high temperatures—often in the range of millions of degrees Celsius—to provide them with sufficient kinetic energy. This energy enables the nuclei to overcome the Coulomb barrier, allowing them to fuse and form a new, heavier nucleus. The fusion of deuterium and tritium, for example, produces helium and releases a significant amount of energy, primarily in the form of kinetic energy of the resulting particles.
One of the critical challenges in achieving nuclear fusion on Earth is maintaining the necessary conditions for a sustained reaction. In magnetic confinement fusion, devices such as tokamaks use powerful magnetic fields to confine and stabilize a plasma of hydrogen isotopes at high temperatures. In inertial confinement fusion, high-energy lasers compress small fuel pellets to achieve the required conditions for fusion. Both methods require precise control and advanced technology to ensure that the fusion reaction can be initiated and sustained long enough to produce more energy than is consumed in the process.
The energy produced through fusion is far greater than that of fission, with estimates suggesting that the fusion of a small amount of fuel could generate energy equivalent to that produced by burning several tons of fossil fuels. This incredible energy density, coupled with the abundance of fusion fuel, makes it a highly attractive option for future energy solutions. However, despite extensive research and investments, practical fusion energy remains elusive, with scientists and engineers continuing to work towards achieving net-positive energy from controlled fusion reactions.
Applications of Nuclear Fusion
Nuclear fusion holds the promise of revolutionizing energy production, primarily due to its potential to provide a nearly limitless source of clean energy. Fusion power plants, once developed, could provide a stable and sustainable supply of electricity without the long-lived radioactive waste associated with fission reactors. The abundant fuel sources for fusion, such as deuterium from seawater and lithium for breeding tritium, could supply humanity’s energy needs for centuries. As the world grapples with climate change and the need for sustainable energy solutions, fusion represents a compelling alternative to traditional fossil fuels and even current nuclear fission technology.
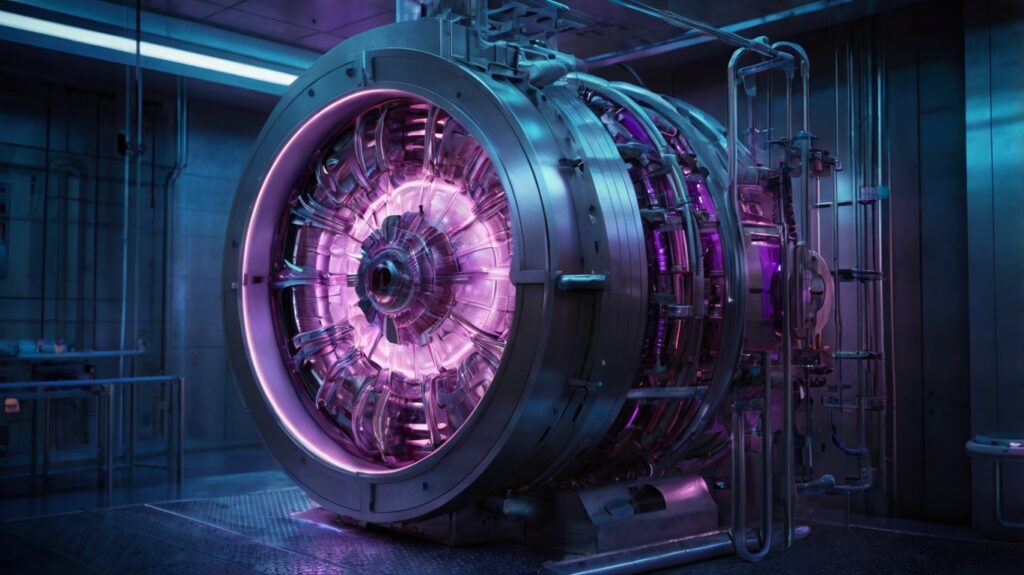
In addition to energy generation, nuclear fusion has potential applications in various scientific fields, including astrophysics and materials science. Understanding fusion processes can provide insights into stellar formation and the life cycles of stars, enhancing our knowledge of the universe. Furthermore, research into fusion materials, such as those that can withstand extreme temperatures and radiation, can lead to advancements in other technologies, including aerospace and medical applications. The techniques developed for fusion research may also have implications for other areas of science and engineering.
Moreover, fusion technology could play a significant role in addressing global energy security. As nations seek to reduce their dependence on fossil fuel imports and mitigate the geopolitical risks associated with energy supply disruptions, fusion offers a path to energy independence. By investing in fusion research and development, countries can position themselves at the forefront of innovative energy solutions, fostering economic growth and technological leadership. The transition to fusion energy has the potential to reshape the global energy landscape, making it a critical area of focus for researchers and policymakers alike.
Advantages and Disadvantages of Nuclear Fusion
The advantages of nuclear fusion are numerous, making it an attractive prospect for future energy generation. One of the most significant benefits is the virtually limitless supply of fuel. With deuterium extracted from seawater and lithium readily available, fusion has the potential to meet humanity’s energy needs for millennia without depleting resources. Additionally, the byproducts of fusion are primarily helium and neutrons, resulting in minimal environmental impact and no long-lived radioactive waste. This makes fusion a cleaner alternative to both fossil fuels and nuclear fission.
Another advantage of fusion is the inherent safety of the process. Unlike fission, where uncontrolled reactions can lead to catastrophic meltdowns, fusion reactions require precise conditions to occur. If containment is lost or the reaction becomes unstable, the process simply stops, eliminating the risk of a runaway reaction. This characteristic makes fusion a safer option for energy production, alleviating some of the public concerns associated with traditional nuclear power.
However, despite these advantages, nreactoruclear fusion faces significant challenges that have hindered its practical implementation. The extreme conditions required for fusion reactions—high temperatures and pressures—are difficult to achieve and maintain. Current experimental reactors consume more energy than they produce, which raises questions about the feasibility of commercial fusion power plants. Additionally, the technological advancements needed to create efficient and economically viable fusion reactors are still in development, and significant investments in research and infrastructure are required to overcome these hurdles.
Conclusion: Comparing Nuclear Fission and Nuclear Fusion
In conclusion, nuclear fission and nuclear fusion represent two fundamentally different approaches to harnessing the power of the atom. Fission, with its established technology and applications, has been a critical source of energy for decades, providing a substantial portion of the world’s electricity. However, it is not without its challenges, including safety concerns, radioactive waste, and the potential for proliferation. As countries work toward cleaner and more sustainable energy solutions, fission remains a contentious yet vital aspect of the energy landscape.
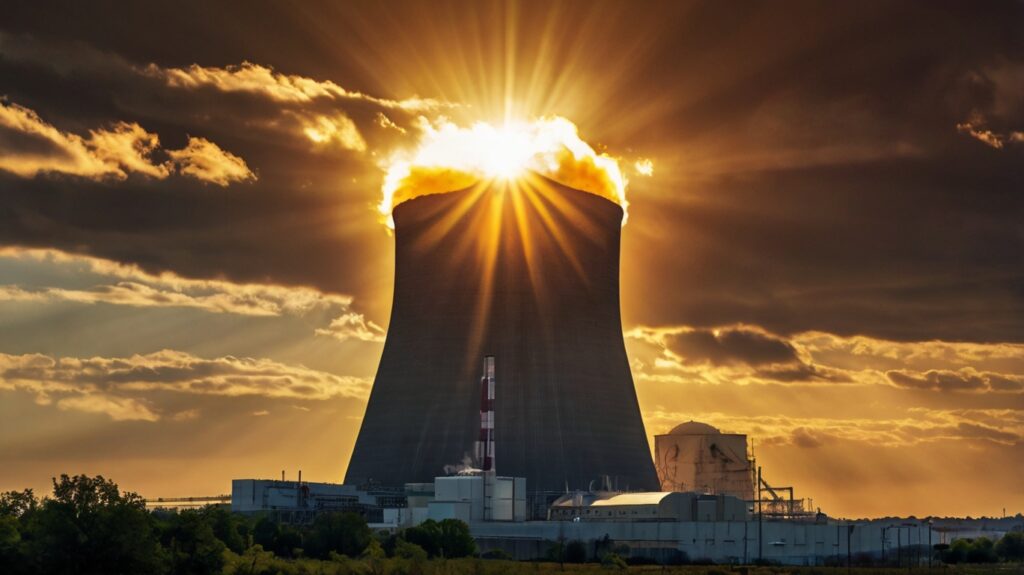
On the other hand, nuclear fusion presents an exciting and potentially revolutionary alternative. With its promise of abundant fuel, minimal environmental impact, and enhanced safety, fusion energy could reshape the future of energy production. However, achieving practical fusion power remains an ongoing challenge, requiring continued research and innovation. As scientists and engineers explore the intricacies of nuclear fusion, the hope is that this clean energy source will eventually be realized, helping to address global energy demands and climate change.
Ultimately, the comparison between nuclear fission and fusion highlights the complexities of energy generation in the modern world. While both processes have their advantages and disadvantages, the future of energy likely lies in a diversified approach that includes a mix of technologies. By leveraging the strengths of both fission and fusion, along with renewable energy sources, we can work towards a sustainable and resilient energy future that meets the needs of society while protecting the planet.